Research Areas
Applied Physics
Physics, the original interdisciplinary science, has a host of applications in many areas of immediate interest and relevance to society. Ion beam analysis techniques such as PIXE and RBS, based on a 3 MV accelerator, are used to study content and structure of environmental samples and hi-tech devices and materials. Medical physics projects employ atomic and nuclear techniques to measure toxic trace elements in the body in the context of occupational health; a system for assessing bone Sr content is being developed. Atomic and nuclear techniques also underpin the development and application of X-ray analysis methods for geochemical exploration of planetary surfaces, through the MER, MSL, and future space missions.
Astrophysics and Gravitation
Research activities in the astrophysics and gravitation group at the University of Guelph cover a broad range of subjects, including high-energy nuclear astrophysics, the physics and astrophysics of black holes and neutron stars, the dynamics of compact binaries, the design of future gravitational-wave detectors, and fundamental aspects of relativistic gravitation. Much of this work is carried out in the context of the emerging area of multi-messenger astronomy, which combines information carried by electromagnetic radiation (in all bands), neutrinos, and gravitational waves. The group enjoys close ties with Perimeter Institute (Siegel and Yang have joint appointments) and with the Canadian Institute for Astrophysics.
The research activities of Liliana Caballero focus on the role of nuclear reactions, neutrino interactions, and nuclear forces on the synthesis of elements and the evolution and mergers of compact objects (white dwarfs and neutron stars), as well as accretion disks around black holes. These stellar environments exhibit strong gravitational fields and extreme thermodynamic conditions, and constitute a unique extraterrestrial laboratory to probe our understanding of the fundamental forces of nature. Caballero's group provides detailed predictions of signals emerging from these systems, which will be observed by various telescopes, neutrino detectors, and gravitational-wave observatories.

from matter accreting into a rotating black hole. The color scale represents the local temperature.
Huan Yang's research work is also grounded in the fundamental processes associated with gravitational-wave and multi-band/multi-messenger astronomy. The work focuses on the dynamics and gravitational-wave emission of black-hole and neutron-star systems, with the goal to extract specific predictions that will be verified in observations by ground-based and space-based gravitational-wave detectors. The work also aims to exploit these observations to gather information about the population of black-hole and neutron-star binary systems in the Universe, their formation scenarios, and the fundamental physical processes involved during their mergers. Yang's group initiated and continues to perfect the design of high-frequency gravitational-wave detectors to probe neutron-star physics, in an effort to plan for the construction of third-generation detectors beyond LIGO and Virgo.

The orbit of the stellar-mass black hole is perturbed by another stellar-mass black hole nearby.
The research efforts of Eric Poisson cover a range of topics in general relativity, including the perturbation theory of black holes and neutron stars, the electromagnetic and gravitational self-force acting on particles moving in a curved spacetime, and the generation and propagation of gravitational waves. The main focus of Poisson's group is on tidal interactions of compact binaries in the context of gravitational-wave astronomy. The tidal interaction affects the orbital motion and the emission of gravitational waves, and the tidal imprint on the waves can thus be measured. Because the tidal deformation of a body is sensitive to the details of internal structure, gravitational-wave observations will eventually reveal intimate details of the physics of a neutron star, in particular the nature of its equation of state.
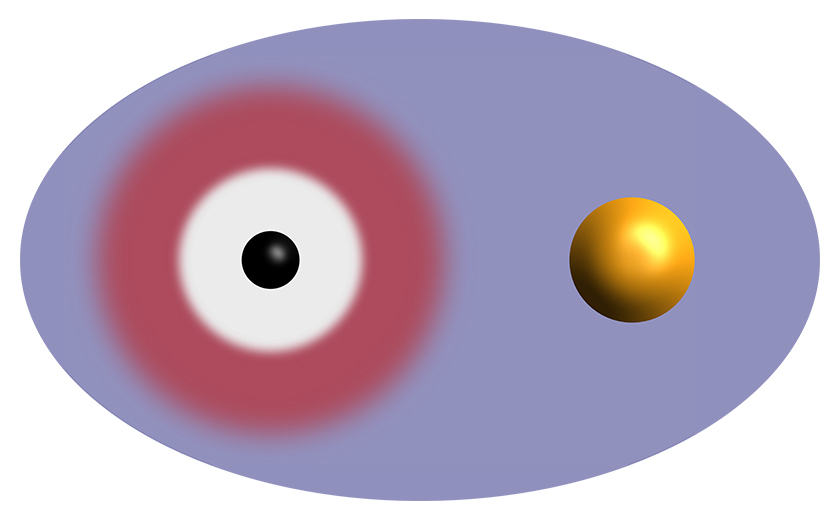
The compact star exerts tidal forces on the black hole, and vice versa.
Atomic, Molecular and Optical Physics
Atomic, Molecular and Optical Physics is a broad interdisciplinary field, with applications in biophysics, medicine, astrophysics, cryogenics, chemistry, environmental science and information processing. Our facilities consist of state-of-the-art equipment, which include some of the world's most intense lasers, a new 3MV accelerator, and the world's first confocal scanning laser microscope, and Canada's only scanning proton microprobe. Many interesting opportunities are also available for theoretical research in this area, and include formal work in variational calculus and boundary value problems, molecular collision theory, ion channel studies, quantum and classical chaos, theory of femtosecond laser interaction with atoms and molecules, coherent control of quantum dynamics, computer simulations of biophysical transport processes, and the calculation of atomic transition rates used in in astrophysical plasma modelling and spectral analysis.
Biophysics
Biophysics is an interdisciplinary science in which the ideas and techniques of physics are applied to gain understanding of biological systems. At Guelph, we study a broad range of problems in biological and medical physics including fundamental aspects of protein structure and function, the physical properties of cell membranes and protein:membrane interactions, assemblies of macromolecules, and non-invasive elemental analysis medical applications. Guelph biophysicists make use of the most up-to-date techniques available, including neutron scattering, nuclear magnetic resonance, X-ray diffraction, vibrational spectroscopy, atomic force microscopy and molecular modeling.
Written by: Michael Stuck & Callum Stonehouse
The University of Guelph’s Department of Physics offers several areas of graduate study in biophysics, including the Photobiophysics Research Group, led by Professor Leonid Brown, and the Protein Nuclear Magnetic Resonance (NMR) Research Group, led by Professor Vladimir Ladizhansky. Both Brown and Ladizhansky have been professors at the university for nearly 20 years.
Brown’s Photobiophysics Group studies light-sensitive membrane proteins. These are proteins within cellular membranes that interact with light to create chemical energy (a phenomenon studied by a branch of biophysics called bioenergetics) or a cellular signal, for example, in vision.
“We use computational bioinformatics techniques to find new, interesting proteins,” says Brown of the work his group does. “Then we make those proteins using microbiological and biochemical methods, and characterize them with all kinds of physical and chemical techniques.”
One such technique that's used is Fourier Transform Infrared (FTIR) spectroscopy, which measures how much infrared light is absorbed by a sample at different wavelengths. The amount of electromagnetic radiation absorbed at each wavelength depends on the molecules in the sample, so measuring the absorption of light can be used to both identify and characterize organic materials.

Professor Ladizhansky’s research group, meanwhile, is most interested in developing NMR spectroscopy techniques[3] to identify organic compounds and explore atomic interactions of biological molecules, getting detailed views of molecular structures. With these methods, the researchers are even able to “see” each atom within the molecule.
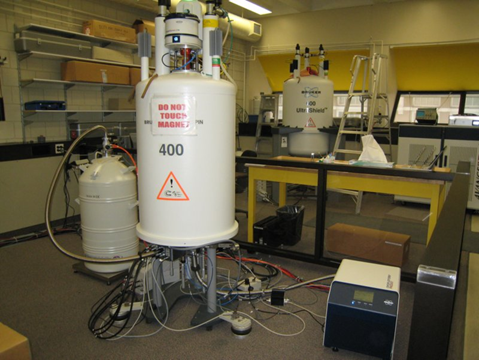
For Ladizhansky, the group’s work embodies why he developed an interest in biophysics, which happened shortly before he came to Guelph. “I became very interested in biology because to me, we are trying to solve problems that are created by nature, as opposed to by humans. I think that it’s much more challenging, and the challenge is what really drives me.”
The research led by Brown and Ladizhansky is highly related, leading to extensive collaboration between the two and their teams.
Recently, both teams brought their skill sets together for a study of membrane protein folding. Folding is a process in which the chains of amino acids that make up proteins fold into well-defined three-dimensional shapes, and the resulting structure is key to defining its function. By understanding how proteins fold—and why they sometimes misfold—we can gain a better understanding of the causes of several diseases. For example, α-synuclein, a protein found in brain tissue, is known to cause Parkinson’s disease when misfolded versions accumulate.
The sequence of steps in the folding process is often quite elaborate, making this an exciting challenge. Working together, Brown and Ladizhansky have made significant in-roads, reporting high-resolution observations of the sequential steps in protein unfolding, which gives some insight into the complex folding process[5].

one of manymolecules which the Biophysics groups study.
(photo: Wang et al; credit to Shenlin Wang and Leonid Brown[6])
Brown and Ladizhansky are both deeply committed to engaging undergraduate students in cutting-edge research. Both professors supervise undergraduate research positions almost every summer, allowing for many research opportunities for students. “We are proud of our record,” says Brown. “I would say that almost every undergrad who has worked with us ended up with their names on published papers, and some on several.” For example, students working with Brown recently discovered new, previously unstudied proteins[7].
Brown and Ladizhansky have found that the wide-ranging research their groups conduct is often attractive to prospective graduate students. “There are many different projects that can be offered to grad students with diverse interests,” says Ladizhansky. “It can be anywhere from quantum mechanics, when it comes to the development of new NMR techniques, all the way to more biochemically oriented projects where you have to express proteins, purify them, and characterize them using a variety of different methods.”
The work being done in this area at the University of Guelph is on the cutting edge of modern physics. “We are trying to do new things which nobody has ever done, both biologically and spectroscopically,” says Brown. “We are trying to go to uncharted territory, because this is what’s exciting.”
Chemical Physics
The interplay between physics and chemistry constitutes one of the most interesting blends of pure and applied research today. Our experimentalists are at the forefront of this research, performing some of the most precise spectroscopic measurements in the world and detecting phenomena as exotic as water on the sun. A variety of advanced techniques are employed in this work, including thin film preparation, Fourier Transform Infrared Spectroscopy, High Resolution Photoionization, Nuclear Magnetic Resonance and Field measurements of atmospheric clouds. There is ample opportunity for theoretical work as well in statistical mechanics, non-equilibrium thermodynamics, the determination of intermolecular forces many-body theory, density functional theory and various mathematical modelling techniques.
Condensed Matter and Material Physics
Condensed matter physics, with its inexhaustible wealth of theoretical concepts and experimental applications, forms the largest branch of physics research today. Our experimentalists probe the most novel properties of fluids, crystals, gels, macromolecules, semiconductors, and metals, in order to determine the collective behaviour of matter under as broad a range of circumstances as possible. Our theorists work closely with their experimental colleagues to understand phenomena as diverse as high-temperature superconductivity, spin-glasses, glass transitions, chemisorption, vortices, surface and interfacial phenomena, structure of polymers and proteins, and critical phenomena. With numerous applications in lubrication, batteries, logic circuits and plasmas, our investigations into condensed matter present students with perhaps the broadest range of career alternatives in physics.
Nanoscience
A dramatic transformation in science and technology is happening. The next fifty years will see new inventions, novel products, stunning medical advances, remarkable energy solutions, and creative answers to controlling and understanding technological and biological processes - and nanoscience is making them all possible. Our nanoscience researchers use a wide range of state-of-the-art experimental and computational techniques for studies of matter on the nanoscale, ranging from the self-assembly of polymers to the optical properties of single sheets of graphite called graphene to the mechanical properties of unique biomaterials derived from bacteria. Exciting opportunities exist for students to get involved in graduate research as well as academic studies in our new B.Sc. Nanoscience degree program.
Written by Michael Stuck, edited by Callum Stonehouse
Nanoscience research explores physical processes on the nanometer scale. At the University of Guelph, nanoscientists in the Department of Physics probe polymeric materials, biomaterials, and graphene on these incredibly small scales, providing unique training opportunities through cutting-edge work led by faculty members such as Dr. John Dutcher.
Dr. Dutcher’s path in physics has been driven by his curiosity to learn new things: “The beauty of science really is all about learning and continuing to learn new things; it’s what we should all strive for,” he says. Dutcher and his team are currently focused on two main research projects: the study of an organic nanoparticle called phytoglycogen and the study of polyethylene pipes used for water lines.
Phytoglycogen is a nanoparticle that was isolated and purified from sweet corn by researchers in the Dutcher lab in 2006. The particle is made up of glucose molecules bonded to form what is called a dendrimer, or a repetitively branched structure. In this form, these molecules have very different properties such as unique bonding interactions with water[1] [2] [3]. For example, the phytoglycogen particles can absorb a large amount of water within the molecule’s branches, which yields a special state of water known as nanoconfined water. Since phytoglycogen is a complex particle, Dutcher’s team employs many complementary observation techniques to develop a comprehensive understanding of this newly isolated substance.
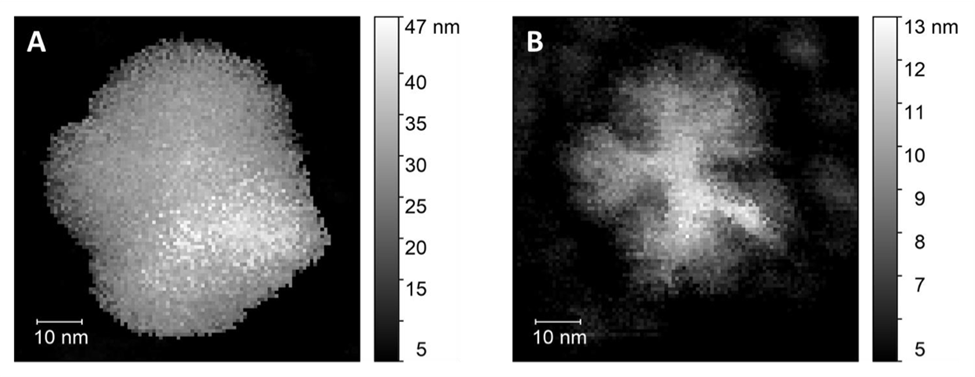
Atomic force microscopy (AFM) height image of a single phytoglycogen nanoparticle measured in water corresponding to the contact point between the AFM tip and the particle; at this height, the nanoparticle appears featureless (left). To the right, a 2 nN deep AFM height image of the same nanoparticle can be seen, with the branching structure of the nanoparticle now visible. (photos: adapted from B. Baylis et al [4])
There are many potential applications for these phytoglycogen nanoparticles in areas such as medicine and personal care[5]. One exciting application is drug delivery, described by Dutcher as, “one of the holy grails of nanoscience.” The unique structure of phytoglycogen makes it attractive for acting as a transport system for medical purposes, particularly since it poses no threat of toxicity to the human body – enzymes just break the nanoparticles down into glucose, the chemical fuel for cellular function. “The thing that really gets me excited about the applications is the biomedical promise for these particles,” says Dutcher.
From drug delivery to our communities’ water supply, the Dutcher lab is exploring innovative ways to improve our world! In addition to nanopharmaceutical research, Dr. Dutcher is excited about applying his decades of experience in polymer physics to the use of polyethylene pipes for industrial and domestic water lines. This project is a terrific example of academic collaboration with industry, with the research funded by a Calgary-based company that manufactures and distributes plastic pipes in place of copper. Using infrared spectroscopy and infrared microscopy, Dutcher’s team analyzes the pipes and tracks what happens to them over time, using heat to accelerate the aging process. The team’s goal is to understand how the pipes behave when exposed to both water and air in order to assess their long-term stability[6].

In addition to leading a busy research lab, John Dutcher is the Director of the Nanoscience Program at the University of Guelph, which began in 2008. This unique major is closely related to the undergraduate physics program, but with a much greater laboratory/hands-on emphasis. Dutcher notes that because of this lab experience, “I have been able to hire second-year nano students and they are immediately helpful in the lab because they already know how to use all the equipment”. The addition of this program at the University of Guelph is a sign of growing interest in the study of nanoscience and nanotechnology that will only expand in the coming years. Nanoscience has the potential to help advance our world in fields such as health care, energy, and many others. John Dutcher and the Dutcher lab, as well as other nanoscience researchers at the University of Guelph, are always striving to make these advancements possible.
Physics Education
Our faculty members with a particularly strong interest in Physics Education are involved in a variety of activities and projects. These include participation in curriculum development at the University of Guelph, as well as at the provincial and national levels. There is also interest in exploring and evaluating new interactive lecturing methods, developing effective lecture demonstrations and laboratory experiments, using sports and music in physics teaching, creating teaching materials for elementary school teachers, and giving physics-demonstrations presentations to the general public and school groups in person and on television.
Written by Michael Stuck, edited by Callum Stonehouse
The Department of Physics at the University of Guelph has a national reputation of excellence in teaching, in part due to our strong commitment to advancing our understanding of the craft through physics education research. Faculty members Drs. Martin Williams, Joanne O’Meara, and Mike Massa lead the work in this area, focusing on improving the quality of teaching physics and science at not only the university level, but at the elementary and high school levels as well.
One area of research conducted by this group focuses on studying female under-representation in physics. Ph.D. candidate Eamonn Corrigan is currently analyzing data from provincial ministries of education from across Canada—in his own words, “… trying to understand which demographic factors interact with gender to lower enrolment rates in high school physics.” Eamonn is also analyzing Ontario Universities’ Application Centre (OUAC) data to determine the factors that link to student enrolment in undergraduate physics programs in the province. Having a better understanding of the current landscape, the next stage of his work will be to develop and implement effective intervention techniques to address the under-representation of women in physics head-on. This research is conducted in close collaboration with Chris Meyer, an award-winning high school physics teacher in the Toronto District School Board and the current president of the Ontario Association of Physics Teachers.
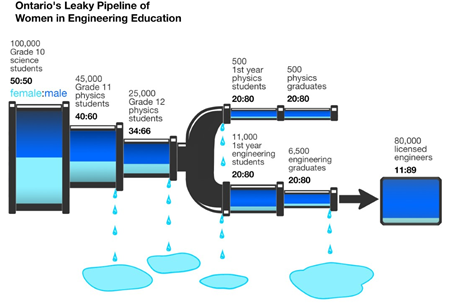
In order to attract bright and critically thinking students to physics, we need to continue to emphasize the relevance of a physics degree to students of all demographics. Corrigan argues that, “As our world keeps developing, the problems we are trying to solve are more challenging, they’re more difficult… I think a physics degree is an excellent way to build a unique set of problem solving and critical thinking skills that can apply to a huge range of future career paths.” As our world changes, physics education has to change as well: it’s this continual assessment and improvement that is the focus of much of the physics education research at the University of Guelph. This research helps to keep the curriculum dynamic, making changes in response to needs and opportunities.
One of these recent changes is the addition of a new third-year course: IPS*3000, Science Communication. The course has been developed and taught by Dr. O’Meara, with the primary purpose of enhancing students’ abilities to convey scientific ideas through various media to a variety of audiences, from younger students to fellow peers and scientists. Communication is an essential skill, especially for students pursuing a career in science, yet it is often left out of physics curricula. IPS*3000 is all about communicating physics and science to non-technical audiences, which is an invaluable skill for physics students to have. Dr. O’Meara’s course ensures that Guelph’s students graduate with practical experience and a clear understanding of how to communicate science knowledge to diverse groups, which gives them an edge in the job search marketplace.
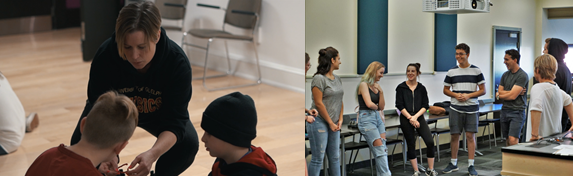
Recent developments have also been made in Guelph’s undergraduate physics program relating to the research of PhD candidate Matt Steffler, who is focusing on integrating computation into the curriculum. These computational techniques are not taught through a dedicated course but are integrated into existing physics courses to promote the relevance of computation and enhance the students’ learning of physics. Through this research, Steffler hopes to continue to further the connection between students learning to code and gaining a better understanding of modern physics. Fundamental programming skills are also very relevant for a variety of career paths. Steffler argues that, “A [basic] understanding of coding is becoming pretty much essential for any technology-based job.” Steffler is working with Drs. Massa and Williams to carefully scaffold the development of key coding skills through assignments and data analysis in almost all of the core undergraduate physics courses.
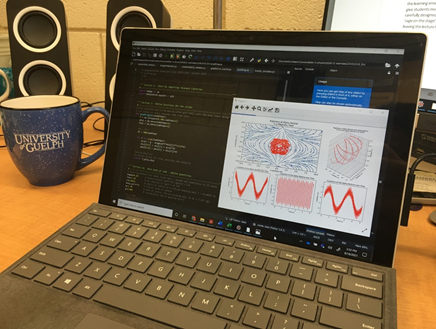
Continual advancement in pedagogy is the hallmark of our approach to physics education at the University of Guelph, and not just in our upper-year core courses. Dr. Williams is a champion of active learning in our large-enrolment first-year courses, where he enhances the learning environment through the development of new labs and in-class activities to give students more ownership over their studies. Active learning engages students with carefully designed discussions and group problem-solving exercises, stepping away from the ‘sage on the stage’ approach. Research has shown that active learning leads to students leaving the lecture hall with better understanding and retention of core concepts.

The University of Guelph’s physics education research group is dedicated to finding ways to improve the quality and relevance of an undergraduate physics degree through encouraging people of all backgrounds to consider studying physics, by focusing on the development of relevant and useful skills such as communication and computation, and by engaging all students in the learning process. With award-winning teachers and dedicated education specialists, the undergraduate physics program at the University of Guelph is respected across Canada for our student-centered focus and commitment to excellence, a guided evolution based on what we learn from the latest research findings.
Planetary Surface Exploration
Faculty member Ralf Gellert is currently the lead scientist for the Alpha-Particle X-ray Spectrometer (APXS) on board the Mars Exploration Rovers (MER). Together with a team of scientists from the Max-Planck Institute in Mainz, the Jet Propulsion Lab and NASA we are performing the daily operations of the instruments on Mars and analyzing the returned data. The APXS is one of the analytical instruments mounted on the rover arm. It measures the chemical composition of rocks and soils with x-ray spectroscopy. Since the successful landing on Mars in January 2004 the rovers Spirit and Opportunity returned more than 100 APXS measurements from each site along their traverse of nearly 5 kilometers. Results of the APXS on both landing sites contributed to the findings of MER that water played a major role during the formation of the encountered rocks and soils. In collaboration with the group of Prof. Iain Campbell we are improving the theoretical model for the data analysis. The APXS uses alpha particles and x-rays from radioactive sources to excite characteristic elemental radiation. The GUPIX package for PIXE (Proton induced X-ray Emission) will be extended to include both of these excitation modes.
Written by Michael Stuck
The University of Guelph is playing an important role in the exploration of Mars. One of NASA’s current missions on Mars, the Mars Science Laboratory (MSL), involves the rover known as Curiosity, which features a scientific instrument developed by University of Guelph Professor Ralf Gellert.
The instrument is called the Alpha Particle X-Ray Spectrometer (APXS) and is one of 11 scientific instruments onboard the Curiosity rover. Ralf Gellert is the Principal Investigator for APXS and leads a team of scientists from the University of Guelph, NASA’s Jet Propulsion Laboratory, and other institutions.
Professor Gellert’s involvement with APXS precedes the MSL Mission, dating back to 2001 and his work on the earlier version of the instrument. At the Max-Planck Institute for Chemistry in Mainz, Germany, he worked as the lead engineer for APXS, which was selected for deployment on the Mars Exploration Rovers (MER), named Spirit and Opportunity. Gellert and a small team built the device and developed the calibration and analysis software. Gellert led the MER APXS operations and data analysis from 2005 until Opportunity, the longer standing of the two MER, stopped working in 2017. He also led a successful proposal to NASA for a new APXS for the MSL. In 2005, after the department that Gellert worked in closed, he came to the University of Guelph where he continued his work with the ongoing MER missions and built a lab to develop and test the new version of APXS for Curiosity.

Curiosity launched in November 2011, equipped with the new APXS model, and successfully landed on the surface of Mars in August 2012. Since Curiosity’s landing, the Guelph APXS team has been responsible for the daily operation of the device, collaborating with the scientists operating the other 10 instruments onboard Curiosity to determine what to do next. Gellert explains, “Each day, we are discussing with the whole science team and the rover planners, what to do next. This involves ‘That rock looks interesting, let’s drive over there’, then there is a discussion of ‘ok, is it worth the resources or could we drive somewhere else?’”. Once a decision is reached, measurements are taken and data are collected, which are then used to inform future decisions.
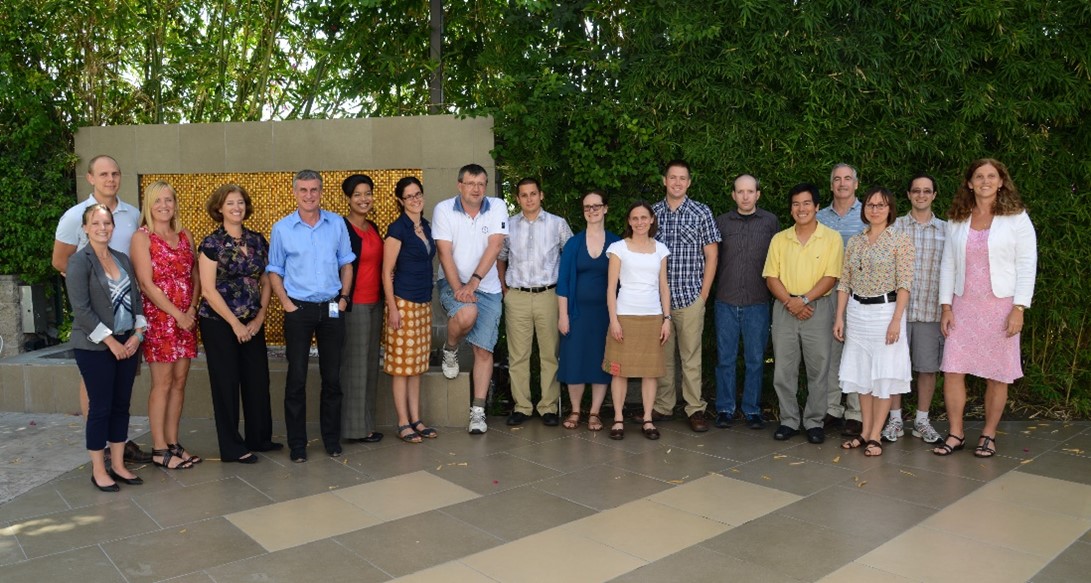
The APXS is used to measure the chemical composition of Martian rocks and soils. It is located on the robotic arm of Curiosity so that it can be placed near, or in contact with, samples of interest. The APXS works by bombarding the sample with radiation (alpha particles and X-rays), which can knock tightly bound electrons from the atoms within the sample, creating a vacancy. When the vacancy is then filled by another electron, an X-ray is emitted in the process. These X-rays are unique signatures for each element in the periodic table, allowing the APXS to determine the types and abundance of elements in the sample.

The APXS team is focused on giving precise concentration values to geological experts for interpretation. The team is constantly working to improve their ability to produce more reliable and accurate values. Gellert explains, “our scientific goal for the APXS team consists of trying to extract all possible information from data we get from Mars. This means if we know we can measure 16 elements, can we measure even more? We see trace elements that are not that abundant in terrestrial samples that we calibrated with. Can we use the data in ways that we didn’t even think about at launch?”
There is still a lot to explore on the surface of Mars. The experts working on the MSL mission are investigating Mars in a way which has never been done before, which is a learning process. Opportunities for scientific inquiry have led to many fascinating student projects at the University of Guelph, many of which have been supervised by Professor Gellert. The projects involve trying to analyze and interpret publicly available data from Mars to gain new understandings. According to Gellert, “all these 4th year projects were very interesting. Sometimes you will see stuff that experts have overlooked over the years because there is so much data.”
You can visit Professor Gellert’s website and more details about the APXS are provided by the Canadian Space Agency: https://www.asc-csa.gc.ca/eng/astronomy/mars/apxs.asp
References
https://link.springer.com/article/10.1007/s11214-012-9892-2#Sec26
https://link.springer.com/article/10.1007/s11214-012-9873-5?no
NASA Science Mars Exploration Program. APXS. Retrieved from: https://mars.nasa.gov/msl/spacecraft/instruments/apxs/
Bruker. Handheld XRF: How it works. Retrieved from: https://www.bruker.com/products/x-ray-diffraction-and-elemental-analysis/handheld-xrf/how-xrf-works.html
Subatomic Physics
Subatomic physics is the study of the constituents of matter on the scale of the atomic nucleus, and smaller, and their interactions. Our primary experimental efforts are centered on nuclear structure, nuclear astrophysics, and searches for physics beyond the Standard Model using Canada's world-leading radioactive beam facilities, ISAC and ISAC-II, located in Vancouver at TRIUMF. Major experimental facilities at TRIUMF operated by the nuclear physics group include the TIGRESS and 8pi gamma-ray spectrometers, and we are currently developing the DESCANT array of neutron detectors. Our research also includes experimental work performed at world-leading laboratories worldwide, including the Maier-Leibnitz Laboratory at Munich, the Van de Graaff Accelerator facility in Lexington, Kentucky, and Argonne National Laboratory near Chicago. Our theoretical efforts concentrate on nuclear forces, the structure of nuclei and the synthesis of heavy elements, the physics of superfluidity, the structure of neutron stars, and several related topics.